225Ac is trivalent radiometal and transform by α-emission. 225Ac transforms with a half-life of 10 days through successive α-emissions, thus releasing 4 α-particles of individual energy per transformation. The daughters involved are relatively short-lived. One of those daughters is 213Bi. This radionuclide represents the last steps of the 225Ac transformation chain. Once radiochemically separated from 225Ac in a radionuclide generator system, it is used as a therapeutic radionuclide despite its short half-life of 45.6 min. Actinium-225 has traditionally been obtained from a long-lived 229Th (t½ = 7340 years) of which 233U has been the only viable source. This route provides 44.4–62.9 GBq (1.2–1.7 Ci) of 225Ac world-wide. Recently high-energy proton 225Ac through the spallation of natural Th through the nuclear reaction:
232Th(p,x)225Ac
This route can provide high quantities of 225Ac in short irradiations meeting the amounts required for clinical evaluations.
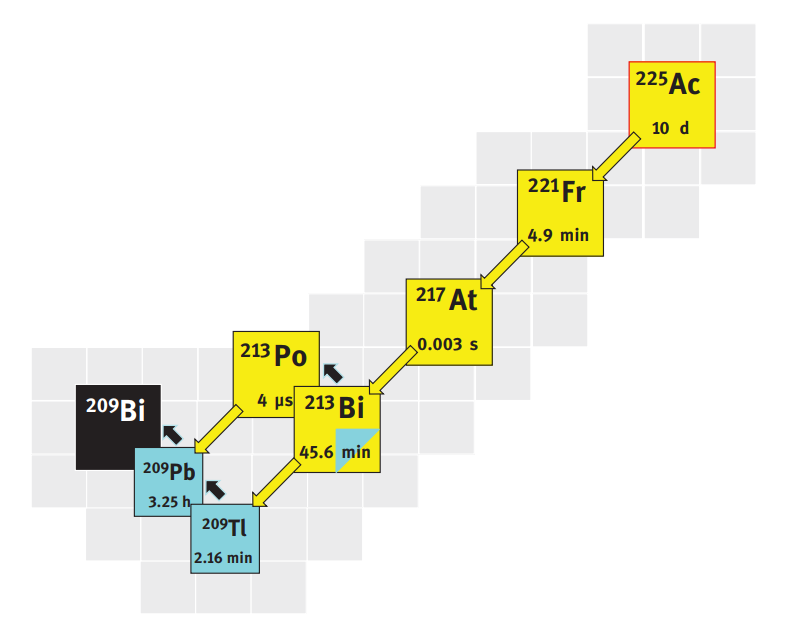
Properties:
Actinium-225 (225Ac), half-life of 9.92 d; 100% α; 5,830 keV 50.7%; 5,792 keV 18.1%, is an alpha-emitter that decays first into Francium 221.
◼ 225Ac emits several gammas, all of them below 1% and the two highest are 100 and 150 keV.
◼ 221Fr (half-life of 4.9 min; 100% α; 6,341 keV 83.4%) decays into Astatine-217. 221Fr emits gamma at 218 keV (11%).
◼ 217At (half-life of 32.3 ms; 100% α; 7,067 100%) decays into Bismuth-213.
◼ 213Bi (half-life of 45.6 min; 100% α – 5,869 keV, 93% – and β- – 1,427 keV, 64.5%, 987 keV, 30.4%) decays in Thallium-209 (209Tl, 2%) and Polonium-213 (213Po, 98%). 213Bi emits gammas at 440 keV (26%). Both 209Tl (half-life of 2.2 min; 100% β-; 1,832 keV 98.8%) and 213Po (half-life of 4.2 µs; 100% α; 8,376 keV 100%) decay into Lead-209.
◼ 209Tl emits gammas at 1,567 keV (100%), 465 keV (97%) and 117 keV (84%).
◼ 209Pb (half-life of 3.25 h; 100% β-; 644 keV 100%) transforms into stable Bismuth-209 (209Bi).
Actinium-225 is the parent radionuclide in the 225Ac/213Bi generator. Other intermediates (217At, 213Po, 209Pb) have either no or below 0.1% gammas, which means that they cannot be detected anymore once injected in man. Maximum specific activity is 58,000 Ci/g.
Toxicity: Unbound 225Ac concentrates in bone and liver.
Manufacturing, sources and availability:
There are five different ways to produce 225Ac that all have been explored so far:
1. Purification from Uranium-233 – The Thorium/Actinium generator
225Ac has historically been produced at an annual volume of between 600 and 800 mCi of carrier free 225Ac through the natural decay of Thorium-229 (half-life 7,340 years) itself decaying from Uranium-233. The extraction process of 229Th could be performed on larger amounts of 233U (half-life 160,000 years). The majority of the 233U stockpiles was produced between 1954 and 1970 via neutron irradiation of 232Th. DoE has a few 100 kg stocks of
233U (some other sources speak about 2 tons) but this is strategic material and only a few Ci of 229Th are available presently for decay product extraction. However, to be efficient the extraction process can only be repeated once every 30 years, making it cumbersome and costly. In average 1 mCi 229Th can generate in theory 7 mCi 225Ac per year and 187 mg of 229Th can be extracted from one kilogram of 233U. The specific activity of pure 229Th is 212 mCi/g, meaning in other words that 1g of pure 229Th can generate about 1.5 Ci/year 225Ac in theory. The material used presently in generators is far from reaching 100% of specific activity.
In 2005, the U.S. Congress ordered the DoE to cease extraction of 229Th from 233U stockpiles and to instead begin down-blending (dilution with 238U to a non-weaponizable 233U concentration) and permanent disposal of the two tons of stockpiled 233U. Completion of these efforts was scheduled for 2018, but only half of the 233U inventory at ORNL had already dispositioned by 2017 (the one not requiring downblending). The next step will not be completed before end of 2024. During this process the 229Th will be extracted by the company Isotek and transferred to TerraPower (see below).
Sources:
◼ Present ORNL capacity for manufacturing of 225Ac based on 229Th decay (the 229Th/225Ac generator) is estimated at about 700 mCi/year. During the years 1991-1992, 90 mg of 229Th with a specific activity of 0.36% were recovered. An additional 50 mg with a specific activity of 24.4% were recovered in 1998-9. This product is
contaminated with some 228Th but no actinium decayed product is obtained from this isotope. These amounts were split in four fractions at ORNL and allow producing up to 700 to 900 mCi 225Ac a year which is sold presently to 6-12 different companies. By March 2018, ORNL announced that this production was subcontracted to an undisclosed private company for sales. It appears that this corresponds to the TerraPower project (see below). A DMF for 225Ac was filed by DoE at FDA in February 2020, but there is no program of increasing the capacity at
ORNL through this route. In April 2021, DoE filed a DMF at the FDA for this quality of 225Ac.
◼ One other important source of 225Ac is IPPE in Obninsk, Russia which can presently produce about the same amount of 700 mCi a year, following the same process, from a source of 150 mCi (704 mg) of 229Th. Information about access to additional 233U in Russia remains confidential.
◼ The Institute for Transuranium Element (ITU) in Karlsruhe, Germany is also producing 225Ac together with the decay product 213Bi for its own research program for alpha-labeled antibodies but in small amounts (however, this still represents about 300 mCi/year). This generator is based on a stock of 229Th of 45.6 mCi (or 215 mg) obtained from ORNL. Each cycle of production (6 cycles a year in 8-9 weeks interval) leads to about 45-50 mCi of 225Ac. Very recently ITU decided to make more 225Ac available for direct labeling rather than transforming it in 225Ac/213Bi generators.
◼ Canadian Nuclear Laboratories (CNL) does have a stockpile of about half a kilogram of 233U which could lead to a maximum production capacity of 1 mCi of 225Ac. There are presently no commercial plans to extract this 225Ac at CNL. In total the present yearly worldwide capacity from a thorium generator is about 1.7 Ci of 225Ac. ORNL estimated that with an investment of about US$ 100 million in generators the center could increase its production up to 4 Ci per 10 days, so about 120 Ci a year. Of course, there is a similar potential of increasing capacity at IPPE.
◼ TerraPower (USA) obtained access to 45 g of 229Th and with additional raw material could be able to produce about 200 Ci of 225Ac per year with a capacity increase over the next 10 years. The first mCi of raw 225Ac became available by end of 2019. In November 2019, Isotek Systems, TerraPower, and the U.S. Department of Energy (DoE) announced an innovative public-private partnership agreement to produce 225Ac. Isotek is the DoE contractor tasked with eliminating the inventory of 233U currently stored at the ORNL. Isotek will extract and provide 229Th to TerraPower for the production of 225Ac.
◼ Recently the Institute for Energy Technology (IFE), Norway, expressed interest in developing and installing a 225Ac supply chain without providing details about the technology. So far, this must just be considered as a potential development program, but nothing has been implemented and there is no information available confirming the technology. Among independent worldwide distributors next to DoE and IPPE through JSC Isotope,
there is mainly one company that covers the market, ITM (Germany) which relies on the ITU and IPPE sources.
2. Neutron irradiation of Radium-226
The thermal neutron irradiation of 226Ra is another production route of 225Ac, [226Ra(3n,2β)229Th] → 225Ac, but it leads to a mixture of 229Th (half-life 7,340 years) and 228Th (half-life 1.9 years). In order to improve this ratio, an eight-year cooling period is required, complicating also the process. With these processes, 225Ac is produced as
carrier free, however, the purity of the final product is not that good as 229Th always contains some 228Th, generating by-products. Also, this process will be limited by access to 226Ra.
The use of fast neutrons is another way that is now explored as well: [226Ra(n,2n)225Ac], but will also be limited in terms of access to 226Ra. As starting material, it is estimated that 257 mg of 226Ra can be extracted from each ton of U2O3 ore, but 226Ra is already available at some specific stocks in EU, the US and Russia.
3. Spallation of 232Th (target irradiation with protons)
A more efficient method under evaluation starts from Thorium-232 [232Th(p,x)225Ac] but needs at least 100 MeV accelerators which are currently only available at BNL (USA), LANL (USA), TRIUMF (Canada) or INR (Russia). In theory the process could produce up to 300 Ci per month of 225Ac at TRIUMF alone. However, this actinium is contaminated with 227Ac (half-life 21.8 y), 228Ra (5.7 y) which decays in 228Ac (6.2 h), 226Ra (1,600 y), 225Ra (14.9 d) which decays also in 225Ac, 224Ra (3.7 d) and 223Ra (11.4 d). 228Ac disappears totally after a few days. In this process, 225Ra can also be separated from the mixture and the decay of this radionuclide allows obtaining clean 225Ac, unfortunately, at only a 10% level (about 30 Ci/month) of the expected initial target. If a large amount
production is based on this separation, it will generate huge amounts of radioactive waste (90% of non usable material) that will lead to very high costs, making this process not financially attractive. Nevertheless, this is one of the three methods that allow obtaining (227Ac-free)-225Ac (next to the thorium generator and the 226Ra gamma irradiation with Linac or Rhodotron).
Sources:
◼ Since 2011, LANL, Brookhaven BNL, and ORNL (the Tri-lab Actinium-225 Research Collaboration) have worked together to develop a technology for fullscale production and stable supply of 225Ac based on high-energy accelerator production by irradiating Thorium-232 target foils [232Th(p,x)225Ac] with 100 MeV protons. Ten days irradiation of a 232Th target of 5g/cm² can theoretically lead to 1.5 Ci 225Ac. However, this product is also contaminated with 140La and 141Ce which are quite difficult to separate and it contains at least 0.2% of 227Ac. With the new technology, routine production was supposed to supply more than 100 times the previous annual capacity, expecting about 500 mCi/day compared to the presently routine weekly capacity of 5 mCi. The maximum capacity reached so far was about 180 mCi and by October 2018 a total amount of 1,800 mCi had been produced among which 235 mCi have been distributed to end-users (corresponding to 15 batches). The advantage of the manufacturing process is in the 232Th handling, 232Th being a low radioactive material compared to 226Ra, which is considered as another starting material. The project is running smoothly in parallel to the production of generators of 213Bi. Very high-capacity has not been reached, but the aim is to produce several tens of curies per year.
◼ In September 2016, TRIUMF announced a new program of production of 225Ac based on the ARIEL (Advanced Rare IsotopE Laboratory) facility (520 MeV cyclotron) that should allow the production of several curies and up to 100 Ci of 225Ac a year. In fact, the scaling up potential is presently (2020) of 1.3 Ci per month based on three 10-days irradiation (first production in October 2019 in collaboration with CNL at Chalk River for the target processing). In theory the capacity of the tool could allow the production of more than 2,000 Ci per year, but this is not realistically achievable as the target could not survive this amount of energy and all calculations limit to a more realistic 500 kW beam instead of 5,000 kW. In December 2021, TRIUMF has entered a collaboration with Fusion
Pharmaceuticals. Fusion will provide a financial investment that will enable TRIUMF to upgrade its production infrastructure. In return, Fusion will gain preferred access to actinium-225.
◼ INR in Troisk (Russia) operates a 160 MeV with up to 140 µA current accelerator which irradiates also 232Th. It has a present maximum capacity estimated at 27 Ci per year. In fact, the process takes 10 days irradiation followed by 10 days decay. In theory this linac could produce up to 3 Ci of 225Ac every two weeks, but the site is not equipped to operate when external temperatures are too high and therefore, the site cannot be used for routine production.
◼ The US company NorthStar Medical Radioisotopes is developing a 225Ac production process also based on the proton irradiation of Thorium. The company has explored several routes based on DOE legacy material and is presently examining high energy proton spallation to produce both 225Ac and 225Ra. NorthStar is performing its production at the Fermi National Accelerator Laboratory (FNAL) 8 GeV booster synchrotron at 400 MeV. The company’s core expertise is brought in the separation technology. In 2019 the expected production amounts was
supposed to reach the 100 mCi per run, but so far, NorthStar just confirmed they are on track.
◼ The Arronax center in Nantes France with its 70 MeV cyclotron is exploring this route, but its theoretical yearly capacity will be limited to about 12 Ci. The lower current electron accelerator 50 MeV, 10 µA ALTO in Orsay, France could also theoretically produce up to 1.5 Ci 225Ac a month.
◼ The IsoDAR cyclotron project (60 MeV, 10 mA) details also how this tool could produce 225Ac in very high-capacity (200 mCi/h). But this remains a project only for the time being.
◼ In 2021, the Peking University, Beijing, China, reported also the first trials with the CYCIAE-100 accelerator (100 MeV H-, 500 μA) which led to the production of 619 µCi 225Ac per batch.
However, for all the above-described sites, one has to take in account that additionally to each mCi of produced 225Ac, 12 mCi of fission product waste are generated as well. As a consequence, this is not an ideal technology for the future as the CoGs are quite high due to these large amounts of useless and long half-life radioactive products generating high cost for waste elimination.
Eventually, contaminants could be eliminated by quantitative mass spectrometry separation techniques, but this will become quite expensive. In fact, both the Isotope Separator and Accelerator (ISAC) at TRIUMF coupled with Isotope Separation On-Line (ISOL) and the ISOLDE (Isotope Separator On-Line Device) program at CERN
(Switzerland) based on a mass separation of uranium or thorium targets irradiated by protons, are able to produce small but clean amounts of 225Ac. In September 2018, CNL became associated to this program. However, the capacity of this separation technology is theoretically limited to 10 mCi per month at TRIUMF and 3 mCi per month at CERN. These amounts remain insignificant compared to the real needs of the industry and these tools cannot be developed realistically as large capacity production solution.
4. Accelerator production (226Ra target irradiation with protons or deutons)
Proton beam: [226Ra(p,2n)225Ac] route:
Cyclotron production is possible by irradiating 226Ra targets via [226Ra(p,2n)225Ac] at about 15 MeV, but only a few sites are or were able to produce it routinely so far. Theoretical yield is estimated at around 18 MBq/µA-h. In other words, a 20-hours irradiation at 50 µA will lead to 18GBq (or 486 mCi) 225Ac. In theory, combining available cross-section data with stopping power for 226Ra suggests a single 20 MeV, 500 µA proton beam incident on a 226Ra target (∼1 g) could produce a theoretical maximum of 4 TBq (108 Ci) of 225Ac per month. Additionally, this product will be contaminated with about 11% of 226Ac (half-life 29.4 h) which has the advantage of decaying faster than 225Ac, but will reduce considerably the overall yields and will not allow to obtain entirely pure 225Ac. So, the
process is quite complicated to implement as it needs the preparation and handling of radioactive targets. Also, access to 226Ra with the adequate quality may be limited for the time being.
Sources:
◼ The company Actinium Pharmaceuticals Inc. collaborated with ITG/ITM Munich, Germany, to develop a process of 225Ac cyclotron production in the years 1995-2005, but the cyclotron producing 225Ac at the Munich site has been shut down in 2013. ITM still detains the knowledge and the technology for 226Ra targetry, but no equipment anymore.
◼ The Dutch company Alfarim announced recently their involvement in the production of 225Ac via the cyclotron route. The company announced a yearly production capacity of 5 to 10 Ci, but they have not started their program yet.
◼ KIRAMS in Seoul, South Korea, claims being ready to produce 225Ac by September 2019 using their 30 MeV cyclotron. This was not confirmed yet.
◼ The Czech center of Rez at the Nuclear Physics Institute CAS has built a site equipped with a 24 MeV ACSI cyclotron that was commissioned in 2015. This site intends to produce also 225Ac.
◼ It seems that the 225Ac production in Japan will also be based on this technology and route and 225Ac could be produced within the next years at the site of Shimitomu.
Deuton beam: [226Ra(d,3n)225Ac] route:
Another route that is presently under final stage evaluation needs accelerator produced 15 MeV deuterium beams and is based on the route [226Ra(d,3n)225Ac]. This new way of producing 225Ac seems to become the most explored route principally using linear accelerators rather than cyclotrons. Yields could in theory reach several tens of thousands of curies per year, depending upon the energy of the beam. No industrial process has been built so far, but investment remains below EUR 30 million to complete full development. By-product in this reaction is 226Ac. An estimated 1.5 Ci/10d of 225Ac could be produced from a 100 mg 226Ra target at energy of about 35 MeV which corresponds to about 50 Ci/year. The US company Nusano is the most advanced in this technology. The Canadian company Mevex claims also to have a similar solution for producing 225Ac.
It is to note that in both cases cyclotrons and Linacs, the target remains the radioactive 226Ra which adds additional complication in the handling. However, such targets have been used for several years in Munich without major issue. Also, there are only a limited number of companies that can provide the starting material 226Ra. For the time being, manufacturers of 225Ac have to rely to the DoE (USA), JSC/Obninsk (Russia) and SCK CEN (Belgium).
5. Electron beam and photoconverter production
An electron beam generated by an accelerator impinges on a photon converter to irradiate a target of 226Ra, inducing a photon-neutron reaction to 225Ra, which decays to 225Ac through the route [226Ra(γ,n)225Ra→225Ac]. This technology is based on different types of electron accelerators and does not generate 227Ac as by-product.
◼ Niowave is developing such a technology based on a super-conducting electron linear accelerator. Demonstration-scale production of 10 mCi batches of 225Ac and first data were published in 201933. In between no additional information on this topic was published so far. The commercial-scale system is expected to produce
first 100 mCi per week and then increase up to 10 Ci per week. The radon present in the process needs a storage period of 60 days.
◼ If linac production works well then there is no reason why Rhodotron should not be able to provide the same yields. The Belgian company IBA is now proposing a Rhodotron that should be able to produce 225Ac in large amounts. The first prototype is under construction and cost of the equipment remains below EUR 20 million. However, cost of processing targets is higher than standard due to the need to trap the volatile 222Rn, decay product of 226Ra and adds at least another EUR 20 million to the project. Additionally, access to pure 226Ra is quite expensive as well.
◼ With the acquisition of Rhodotron accelerators in March 2019, NorthStar has now also the possibility to produce 225Ac via the photon process. The company has not announced yet their intention to progress with this route as they have put the priority on 99Mo production, but the contract includes the acquisition of 8 accelerators in total which leaves a good opportunity for the company to develop other radionuclides, including 225Ac.
◼ The company Hitachi in Japan is exploring this route as well (Linear accelerator of Tohoku University) and published the first data in 2020. The authors of the most recent publication (2021), from the NRC and Chalk River
Laboratories, Canada, describing an application of this technology, claim this technology would be capable of producing a yield of about 148 GBq (4 Ci) of 225Ra from a 10-day irradiation of 1 g of radium segmented into two to four separate encapsulated targets at a beam power of 20 kW. These targets could be milked at least three times to produce this amount.
6. Alternative production routes
Other routes such as [226Ra(p,pn)225Ra]→225Ac, [232Th(p,4n)229Pa], [238U(p,x)225Ac], [232Th(n,γ)233U] or [230Th(γ,n)229Th] have been explored but proved to be impractical due to either low yields, limited access to target material or sensitive by-product production. In Japan, 225Ac is available from RIKEN and Osaka University. Nihon Medi-Physics claimed at the 2019 TAT11 meeting that they are setting up a production facility for 225Ac
in Japan by end 2019. Details about the technology are missing.
Needs:
In summary, nowadays, small amounts of 225Ac are available from ORNL, ITU (JSC) Karlsruhe, Germany and JSC Isotopes Obninsk, Russia directly or via distributors such as ITM Medical Isotopes. There are two qualities of 225Ac available one being contaminated with 227Ac and not useful for large scale human use. Larger amounts could become available if investments are made in dedicated linear accelerators.
It is estimated that the average patient dose could stay around 3µCi/kg, so about 300 µCi per patient and per dose will be needed at EOB if we take in account decay in transport and labeling yields. This is about 400 times lower than a standard beta emitter such as 177Lu, but also 20 times lower than the 211At patient dose. As a consequence, for an average 225Ac radiolabeled drug that targets about 100,000 patients worldwide, the yearly
needs will be around 30-40 Ci, but much more if indications are broad ones such as prostate cancer. The radiopharmaceutical industry should target about 200 Ci yearly production overall capacity to guarantee the supply for the major projects under development.
Derivatives:
Apart from some rare research units that have access to the radionuclide, 225Ac-labeled derivatives have mainly been explored by:
◼ MSKCC: 225Ac-J591 – early clinical stage
◼ Actinium Pharmaceuticals: 225Ac-Radretumab – L19, EDB inhibitor – preclinical stage, 225Ac-Lintuzumab (Actimab-A, anti CD33 mAb for Acute Myeloid Leukemia therapy, early clinical stage), 225Ac-Daratumumab (CD38 Multiple myeloma, preclinical)
◼ Fusion Pharma: 225Ac-FPI-1434 (targeting IGF-R1 in solid tumors, Phase I)
◼ University of Heidelberg: 225Ac-PSMA-617 (prostate cancer Phase I/II)
◼ Telix Pharma: 225Ac-TLX251 (CA-IX, kidney cancer, 225Ac-TLX591 and 225AcTLX592 (prostate cancer)
Actually, all molecules presently under development as 177Lu-labeled drugs have a potential to be developed as a second-generation drug labeled with 225Ac. These include derivatives of DOTATATE and DOTATOC or DOTA-SP. Additionally, it seems that the tandem therapy consisting in co-injection of the same vector labeled with 177Lu and 225Ac shows very interesting data.
Besides cancer-related therapies, 225Ac has also been proposed for the treatment of infectious diseases such as HIV. First human trials with 225Ac-PSMA-617 have shown very interesting results, leading in an exploding demand for 225Ac to run similar trials or for compassionate use.
In February 2017, Johnson & Johnson invested US$ 25 million in Fusion Pharma (CPDC) for the development of 225Ac-FP1434. In September 2017, Fusion Pharma secured an additional US$ 21 million in a second closing of its Series A financing and in April 2019 completed a US$ 105 million Series B financing. Their source of 225Ac is unknown, but could probably be related to TRIUMF’s announcement.
225Ac is also used as the parent isotope in the 225Ac/213Bi generator. A commercial (nonGMP) generator for research purposes has been available since 2010 at ITM Munich for research purposes. ITM did run an older cyclotron able to produce small amounts of 225Ac, but this production was stopped and the cyclotron was dismantled in 2013. ITU is now producing these generators, but they are high demanding in terms of amount of 225Ac and in absence of high production capacity of 225Ac, the access to 225Ac/213Bi generators will remain limited and the price of these generators will rise.
Price:
Precise prices for 225Ac are depending upon the source and the regularity of the manufacturing and must be considered only as local production. About 1.7 Ci 225Ac is presently available annually but this capacity is growing. It is estimated that ORNL is making US$ 1.8 million a year with the sale of their 225Ac from the thorium generator. If we consider their yearly capacity at 600 mCi, this brings the mCi bulk price at US$ 3,000.
However, the official price of bulk non-GMP 225Ac solution remains above US$ 7,000 per mCi and even if some other sources can provide it for less than EUR 5,000/mCi, these prices continue to rise. The price for GMP quality will be much higher as soon as available.
Knowing the complexity of the process, it is expected that the price of the radionuclide will probably not drop below EUR 6,000 (US$ 7,200) per final patient dose (about 200 µCi, based on an average dose of 3 µCi/kg) when larger amounts will be available, if still based on the spallation, generator or cyclotron production. Linac production being more efficient, it would not be surprising to see the bulk price of 225Ac dropping below EUR 5,000/mCi, so below EUR 1,000 per patient (CoGs). However, the technology is far from having proven its industrial feasibility and transformation in GMP grade, then pharmaceutical grade is not taken in account in these figures.
Issues:
◼ Worldwide capacity:
➢ There are only a limited number of sites presently able to produce this radionuclide. The global production issue will be solved if the 232Th route is successful, and the 500 mCi/day capacity target will be reached at the time a
225Ac (or 213Bi) labeled drug comes onto the worldwide market. If the patient dose drops below 200 µCi, this daily capacity could represent more than half a million patients a year. Some further manufacturing investments will be
necessary and the Linac or Rhodotron technologies may be the best solutions.
However, additional capacity will be needed if some programs are developed on the basis of 213Bi and will need larger capacity of 225Ac/213Bi generators.
◼ Access to the precursor 226Ra:
➢ There may be an issue with the access and handling of pure 226Ra, the starting material in some processes. Additionally, access to 226Ra is under strong competition with the production of 223Ra as 226Ra is used also as starting material for producing this radionuclide. For its 223Ra supply for Xofigo, Bayer has acquired the DoE stocks of 226Ra which is locally irradiated to produce 227Ra decaying in 223Ra.
◼ The impurity 227Ac:
➢ There are two qualities of 225Ac available nowadays: the thorium decay source which leads to clean actinium, and the spallation produced actinium which is contaminated with 227Ac. In the neutron irradiation process, irradiation of already formed 225Ac results also in the production of the impurity 227Ac (half-life 21.7 years, precursor of 223Ra). The level of 227Ac as impurity in 225Ac can reach 0.3-0.4%. The authorities from some countries have already announced that they will not allow the clinical use of 227Ac-contaminated 225Ac. This quality
remains however, useful for the production of 225Ac/213Bi generators.
The issue with the contamination of 225Ac with 227Ac is similar to the issue raised with ca 177Lu contaminated with the long half-life of 177mLu. There is no real risk for the patient but the waste treatment at industrial plants and even more in hospitals will be very strictly controlled by authorities. As an example, if we consider injecting to a patient 5 MBq (130 µCi) which contains 0.2% of 227Ac, i.e., 10 kBq of 227Ac, and if we consider that this patient will release 50% of this radionuclide through natural excretion during the first 24 hours, 5 kBq will reach the hospital waste tanks. The maximum tolerated amount in waste tanks is 10 Bq/L. In other words, the volume of the tank or the dilution level must at least be 500 L per patient and preferably 1 m3 to be on the safe side. This limits considerably the number of patients that can be treated in a single place.
◼ Decay chain of 225Ac:
➢ 225Ac has a decay chain that includes three other alpha emissions. This is theoretically an advantage in terms of efficacy, but brings additional potential side effects due to uncontrolled biodistribution of decay products. One way to go around this problem will be to trap 225Ac (and the daughter radionuclides) in closed systems such as nanoparticles or nanospheres. For example, it was demonstrated that the encapsulation of 225Ac in polymersomes leads up to 69% retention of 221Fr and 53% of 213Bi. The limited overall toxicity of these decay products may probably lead to an absence of utility of these complex solutions.
The absence of possibility to follow in real time the biodistribution of all alpha emissions could have been a major issue in terms of dosimetry which could be challenged later by the authorities. However, preclinical data generated with 225Ac-J591 and 225Ac-B4 demonstrated clearly the limited diffusion of decay radionuclides 221Fr and 213Bi in mice models treated with internalizing antibodies. Additionally, authorities are usually not requesting data from
individual metabolites (if decay products can be considered as a kind of metabolite) and they want to see toxicology data as a whole, i.e., as a result of the injection of the drug itself. If a metabolite is more toxic than the original
substance, these toxic effects will limit the maximum dose anyway, being radioactive or not. On that basis, i.e., human toxicology data, the compound 223Ra-chloride with the radium showing a similar complicated decay chain, was approved as a drug by the authorities.
Comments:
The decay scheme of 225Ac includes three alpha-particle-emitting daughters. The first promoters of 225Ac (Actinium Pharmaceuticals) used the term ‘nanogenerator’ to describe this radionuclide. The last alpha-emitting daughter in the series is 213Bi. The related in vivo cytotoxicity of 225Ac makes it 1,000 times more potent than 213Bi, and remarkable efficacy had been demonstrated in preclinical studies. Unfortunately, because the chelating group
blocking the actinium to the organic vector will probably not trap the francium, astatine, thallium, polonium or lead (all decay metals from the decay chain), and also as a consequence of the recoil effect, only the first alpha particle may decay in the vicinity of the tumor. Among the radioactive decay products only 221Fr and 213Bi are also gamma
emitters with an emission that can be detected. One must therefore consider 225Ac as a ‘dirty’ radionuclide which generates an efficient first alpha particle, but introduces a toxicity that is three times higher, although probably distributed only in the liver. It could have been expected that the authorities may add some constraints in the clinical use of drugs labeled with 225Ac (healthcare authorities), but also in the handling at the hospital level (waste, staff protection, nuclear safety, i.e., nuclear safety authorities). The decay chain was not a major issue for the authorities (EMA and FDA) who evaluated the first largescale alpha-emitter 223Ra-Xofigo and granted the MA, so it should not be a problem for 225Ac and 225Ac-labeled drugs either.
If decay products may generate side-effects that are stronger than the original alphaemitters 225Ac, these side-effects will just limit the maximum dose to be injected. If efficacy is demonstrated at doses that do not generate side-effects, then it makes no sense to look at the exact origin of these potential side-effects. These properties can lead to the conclusion that 213Bi could be as efficient and should push for more development in this field, providing a 225Ac/213Bi generator is made available.
As alternative to this issue, research programs at MURR have demonstrated that alpha emitters with long decay chains and secondary alpha emissions such as 225Ac or 223Ra can be used trapped in an inorganic crystalline lattice that can be bound to the vector. The Raddel project developing the trapping of radionuclides in sealed carbon nanotubes may become another way to stabilize 225Ac next to the tumor cells whilst also keeping all decay
products in its vicinity. This project is however, on hold.
In these two examples, none of the radioactive decay atoms are released in the organism, keeping the entire radioactivity in the vicinity of the vectors. This could probably be the ideal solution for reducing the additional radiotoxicity due to uncontrolled radioelements or at least to enhance the potential of alpha-emitters by keeping the decay radioactivity in the vicinity of the tumor cell. This approach has to be followed carefully as it may have some
implication in the selection of the next generation of alpha-emitting radionuclides and the needs for specific manufacturing tools. This approach raises other concern namely about the toxicity of the trapping agent (nanoparticles) and for the time being the simple chelating agents should be sufficient to bring alpha-emitter labeled drugs on the market.